WAVES survey is designed to probe the evolution of galaxies and structure over the last ~8Gyr, down to ~106M☉ galaxies and1010M☉ dark matter halos.
WAVES has four key scientific goals:
- probing dark matter
- the low surface brightness and dwarf galaxy regime
- mergers, star-formation and the build-up of stellar mass in galaxies
- the evolution of structure.
Dark Matter
The nature of dark matter is one of the key questions in modern day cosmology. The currently favoured Cold dark matter model (ΛCDM) provides a good description of the large scale structure of the Universe. Comparison of robust model predictions with empirical galaxy clustering measurements on Mpc scales supports ΛCDM for the growth of structure. On sub-Mpc scales, i.e., on galaxy and group scales, baryons and baryonic physics become critical: the kpc to Mpc range is the key scale over which dark matter halos virialize and merge, and baryons decouple, collapse and eventually form complex structures such as galaxies. In this regime, our theoretical understanding is less well-founded, in great part due to the immense complexity of the physics encountered.
Galaxy-group samples are now able to probe down to a few 1012M☉, with arguably the most complete being the “GAMA Galaxy Group Catalogue” (G3C, Robotham et. al. 2011). Properties of low mass galaxy groups are limited by the intrinsic lack of survey depth. This explains why for 1012M☉ systems (Mh12 hereafter), only two well-studied examples currently exist: the Milky-Way and the Andromeda systems, both in our own neighbourhood. Galaxies in the Local Group are extensively used to probe in detail the CDM paradigm. They provide the strongest evidence against the standard ΛCDM model, from the missing satellite problem to the too big to fail problem.
Given that galaxy formation efficiency peaks around Mh12 in all standard CDM models, Mh12 is critical in testing CDM. It is therefore necessary to ensure that this limited sample of well-studied Mh12 systems is representative.
To create such a statistical sample is a central goal of WAVES. The survey depth of WAVES-Wide results in Large Magellanic Cloud-like galaxies to be close to volume limited out to z ∼ 0.2, and is specifically designed to deliver a high fidelity group catalogue (∼4k groups with 5+ members), probing to the very lowest halo masses. This sample size will allow for the intrinsic scatter in the sub-halo / stellar mass occupation statistics to be measured. WAVES-Wide will result in a proper characterisation of Mh12 groups, including assessing how representative our two best studied examples are.

The Low surface brightness and dwarf galaxy regime
The study of the field dwarf galaxy population offers a unique testing ground for galaxy formation and the underlying physics. Cold dark matter (CDM) simulations predict that there are many more low-mass than high-mass halos remaining today. Observationally there appears to be a deficit with respect to this prediction; the observed galaxy stellar mass function is not as steep as the halo mass function. This could be in part because the mass of the dark matter particle is in the keV range (i.e., WDM), which suppresses power on dwarf galaxy scales. However, dwarf galaxy formation is also sensitive to the impact of the photoionizing background, supernovae feedback, and environmental effects. Disentangling the baryonic effects from any change in the power spectrum requires large statistically representative samples.
Local surveys such as the SDSS and follow-on campaigns such as GAMA are fundamentally limited by surface brightness sensitivity of the imaging data, and the reality of the luminosity-surface brightness relation. Various studies clearly demonstrate that the SDSS becomes incomplete for systems with µe ≥ 23.0 mags/sq arcsec which become frequent below absolute magnitudes of Mr = −18 mag. For this reason the low surface brightness and dwarf galaxy domains continue to remain uncharted territory for modern surveys. Only deep surveys of clusters (highly unrepresentative of the average Universe) have entered this dwarf galaxy low-SB regime. The first deep wide-area imaging survey capable of probing into this domain will be VST KiDS covering 750 sq. deg in the Southern and Northern Galactic Caps. WAVES will use the VST KiDS data as its input survey to provide targets to rAB < 22mag with µe < 26 mag/sq arcsec allowing for the construction of a complete sample of galaxies to Mr = −14mag, i.e., 4mags deeper than SDSS. The WAVES survey, rAB < 22, with high-completeness for low-SB galaxies would enable the galaxy stellar mass function to be measured accurately down to 106M☉.
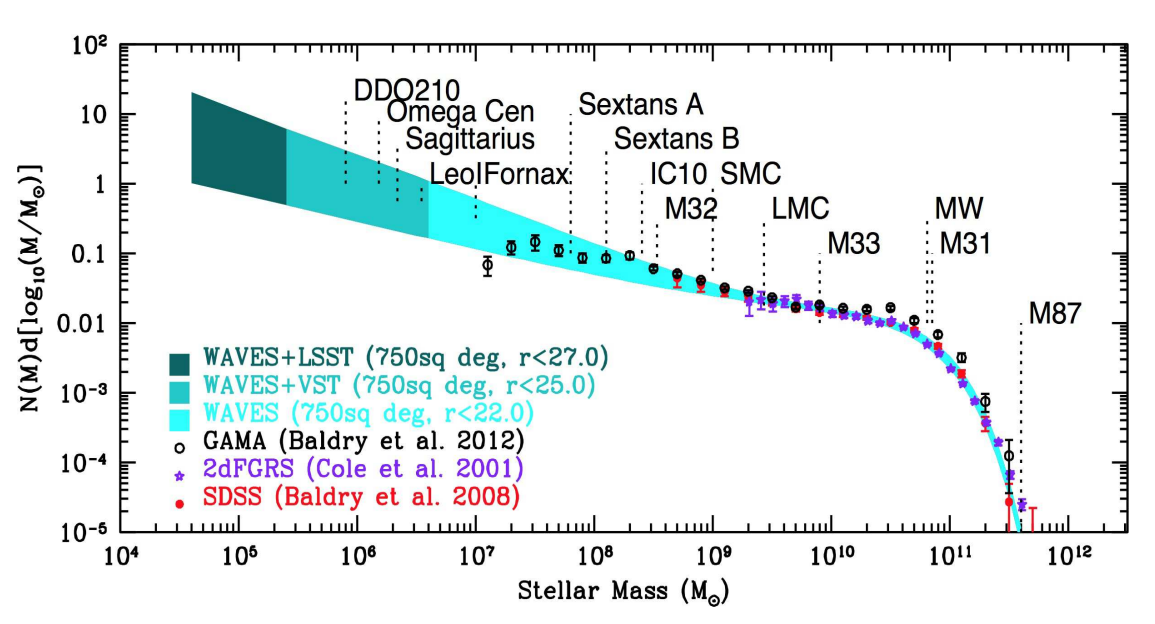
Mergers, star-formation and the build-up of stellar mass in galaxies
Galaxies grow over time, both via the formation of new stars using cold neutral gas and by the merging of multiple galaxy units into a much larger system. These processes are not mutually exclusive, as the merger event can affect the rate at which new stars are formed via complex processes which can either enhance, suppress or completely quench star-formation. In order to probe the buildup of stellar mass in the Universe (a key parameter in understanding the evolution of galaxies), we must study all of these process over a large redshift baseline and for statistically robust samples of galaxies.
Thanks to surveys such a SDSS and GAMA we are beginning to understand these processes at low redshift (z<0.4) and in the intermediate to high stellar mass regime (>109M☉). However, we have little robust understanding of the time evolution of merger rates, consistently measured star-formation in large samples, merger-modified star-formation or how environment affects all of these processes. In addition, from nearby galaxy samples and the local group, we are just beginning to build a picture of how large mass ratio galaxy-galaxy interactions affect star-formation at lowest stellar masses (<108.5M☉), and the likely transition between starvation and ram-pressure stripping quenching in galaxies.
Such low mass mergers may be a critical component of the mass growth and feedback process in galaxies. So far, even GAMA fails to detect these low mass systems outside of the very local Universe. However, in combination WAVES-Wide and WAVES-Deep will probe the factors affecting the build-up of stellar mass in galaxies in both the low mass regime and to high redshift – robustly measuring galaxy merger rates, in-situ star-formation, passive fractions to low stellar masses and merger induced star-formation processes, as well as relating all of these factors to large scale environment. In addition, WAVES-UltraDeep will extend this analysis out to z~1.5, and put the most robust constraints on the merger rate of galaxies over the last 9 billion years.

The Evolution of Structure
Studies of the mass-size relation of galaxies in the nearby (z < 0.1) and distant Universe (z > 1) show a x5 growth in galaxy sizes at fixed stellar mass and in number-density. This extraordinary result, confirmed by numerous groups, implies a dramatic physical change occurring in the galaxy population over the redshift range 0.2 − 1.0. Possible explanations include dynamical relaxation, major mergers, minor mergers, and disc growth.
However, this result is only clearly established for high stellar mass systems (i.e., > 1011M☉), found in extreme dense cluster environments. Whether this growth is endemic or confined to a specific mass or environment remains unclear. A deep spectroscopic survey with HST-resolution imaging over a sustained area is needed to extend the measurements to fainter mass limits and to distinguish between the competing hypotheses.
WAVES-Deep/UltraDeep in combination with Euclid imaging, provides exactly the dataset required to study this extraordinary growth. A key starting point will be to address whether the fundamental nature of galaxies is its bimodality (red v blue), or its evident duality (bulges plus discs). At very low redshift (z < 0.1) this issue is clear-cut with multi-component decomposition a routine part of the analysis toolkit. At high redshift (z > 1.5) the case is less clear as galaxies no-longer appear to adhere to the simple idea of bulge plus disc systems, but exhibit highly asymmetrical and irregular shapes. As such the language of high redshift galaxy work is typically focused on turbulence, distortions, and the global colour (red v blue).
To date the largest contiguous survey by HST covers 1.8 deg2 (COSMOS). Euclid will transform this by imaging upto 8000 deg2 of sky at 0.200 resolution. Surveying this entire area spectroscopically is unrealistic, however WAVES-Deep will provide over 0.5-million galaxies in the range 0.2 < z < 0.8, with imaging resolution sufficient to discern and measure bulge, bar, and disc components to 1kpc resolution, and WAVES-UltraDeep extending this further to 0.5-million galaxies to z < 1.5 in a select number of single pointing fields.
This will open the door for direct measurements of the mass and size evolution of the distinct structural components (bulges, bars, discs) within a sufficiently comprehensive survey to dissect trends by halo mass, star-formation rate or a multitude of other indicators. If tracing the duality of galaxies is critical for understanding galaxy formation, as we suspect, then WAVES-Deep/UltraDeep + Euclid will provide more than an order of magnitude advancement over COSMOS/zCOSMOS, and in doing so firmly bridge the near and far Universe.